This time, we look at two concepts that can massively increase the effective range of particle beam: one is being applied every day in modern accelerators, and the other is an outgrowth of a tool used in biophysics.
Everything here is based on science that has been worked upon by physicists and engineers, and will be referenced when possible.
Performance limits
The key characteristics of a particle beam are its divergence, particle energy and average power. Improving these characteristics leads to increases in overall performance. However, we know that there are limits to the performance that is possible.
One limit is the ion source. The emittance of current ion sources are where they are at today due to the need to vaporize the ions into a gas (which imposes a minimum temperature) and the interactions between ions and energetic electrons or strong magnetic fields (which disturbs the ions).
Expanding the beam to convert emittance into low divergence introduces its own errors and disturbances.
Another limit is the neutralization step. Even when an ion beam and an electron beam are exactly matched in velocity and direction, their recombination into an atom releases energy that kicks particles in random directions. The magnitude of the kick cannot be reduced by any means, so there is a minimum divergence in neutral beams.
Optimizing the beam composition to reduce emittance introduces other problems. The elements that vaporize at a low temperature and have a low ionization energy for their weight (like Cesium) are always heavy. For the same energy added to them, they end up travelling much slower than light elements. For example, 250 MeV added to Cesium only pushes it to travel at 0.063 C while hydrogen atoms of the same energy zip past at 0.613 C. This increases the travel time necessary to reach a target, leading to greater beam spread and a lower hit chance.
Finally, there are the accelerator limitations. For a specific emittance, you can reduce divergence linearly by expanding the beam or speeding it up. Beam expansion becomes more and more difficult in terms of magnetic lens field strength requirements as the beam energy and current increases. Speeding up the beam requires quadratic length increases for each small gain in velocity. Attaining the desired level of performance might lead to gargantuan accelerators.
Beating the laser
The limitations of particle beams are particularly relevant when comparing them to lasers. Both are directed energy technologies that try to cross great distances while maintaining a small beam diameter. A fair comparison between the two would use emittance and divergence.
The neutral particle beams that we can produce today are equivalent in emittance to laser beams with wavelengths of about 100 to 200 nanometers. Lasers struggle to produce these wavelengths efficiently and so particle beams should have the upper hand. However, lasers can work around their emittance by using large mirrors. Just like a particle beam expander, laser optics allow for very low divergence even when using longer wavelengths, such as 700 to 800 nanometers.
Cooled diode lasers are achieving efficiencies of over 80% when producing those wavelengths, eliminating that advantage from a particle beam.
In essence, lasers can trade the complex and heavy equipment needed to beat particle beams on an emittance basis, for the simple and lightweight solution that is large mirrors.
Even better, mirrors can relay the beam over great distances with minimal losses, resulting in a Laser Weapon Web.

The cutting edge of existing technology, paired with beam expanding optics and the use of heavy ions, could produce neutral particle beams with a divergence of 1 to 10 nanoradians. Equivalent laser beams of 1 to 10 nanometers can only be produced by an X-ray Free Electron Laser (XFEL). Comparing between advanced particle beams and XFELs is more nuanced.
XFELs use the same accelerator technology to create a high energy stream of electrons. The electrons can be recycled many times and their energy recovered, leading to efficiencies perhaps greater than particle beam accelerators. At the ranges where an advanced particle beam and an XFEL are effective, light lag is significant. Lasers win out here again as they are many times faster than heavy neutral beams.

However, XFELs have their own unique challenges. They need an undulator to convert the energy of their electrons into photons. It can only extract 0.1% of the electron energy with each pass. Recently, we have devised solutions, such as tapered undulators, that extract up to 10% of the electron energy. This is far below the 100% utilization of a particle beam, so while they may have a 10 to 20% advantage in efficiency, they could end up with a 10 to 100 fold penalty to power density. In other words, for the same mass budget, a spaceship is likely to output much less beam power when using an XFEL than when compared to using a particle beam.

X-rays are very hard to manipulate. There exists no mirror that can reflect them and no lens which can focus them.
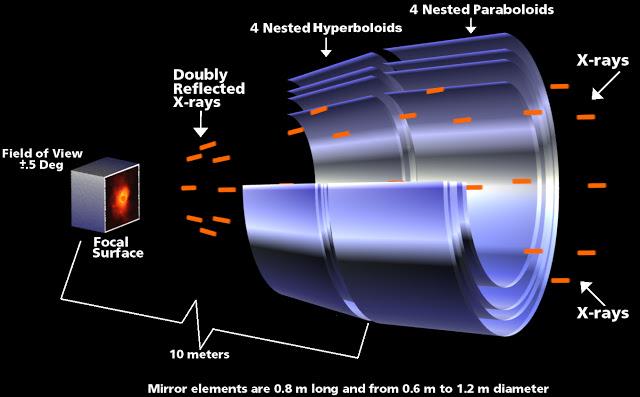
An XFEL would have to rely on grazing-incidence optics to aim and focus its beam. Grazing-incidence optics are nestled cones of a dense metal like gold angled at 1 degree or less.
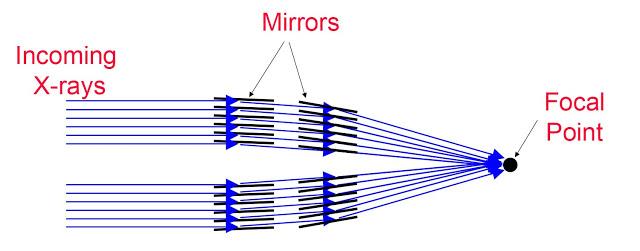
Such an optic would need many, many such cones even at small sizes. As the focal distance increases, the angle of the cones must decrease. A 1 meter diameter optic focused at a spot 10,000km away requires cones of an angle 2.86 *10^-6 degrees. This leads to optics composed of no less than millions of cones, which is incredibly impractical and necessarily heavy. It should also be noted that grazing incidence optics cannot adjust their focal point.
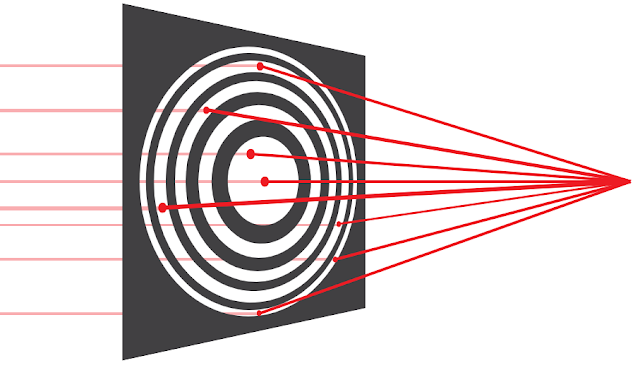
The alternative is a Fresnel Zone plate. It is not as delicate or as intricate as a grazing incidence optic, can adjust its focus and can be a rather lightweight device even when several meters wide. The ‘catch’ is that it absorbs between 50 and 75% of the laser beam.

The plate also needs to be actively cooled and reduces the efficiency of an XFEL far below that of a particle beam accelerator.
The cutting edge of existing technology, paired with beam expanding optics and the use of heavy ions, could produce neutral particle beams with a divergence of 1 to 10 nanoradians. Equivalent laser beams of 1 to 10 nanometers can only be produced by an X-ray Free Electron Laser (XFEL). Comparing between advanced particle beams and XFELs is more nuanced.
XFELs use the same accelerator technology to create a high energy stream of electrons. The electrons can be recycled many times and their energy recovered, leading to efficiencies perhaps greater than particle beam accelerators. At the ranges where an advanced particle beam and an XFEL are effective, light lag is significant. Lasers win out here again as they are many times faster than heavy neutral beams.
However, XFELs have their own unique challenges. They need an undulator to convert the energy of their electrons into photons. It can only extract 0.1% of the electron energy with each pass. Recently, we have devised solutions, such as tapered undulators, that extract up to 10% of the electron energy. This is far below the 100% utilization of a particle beam, so while they may have a 10 to 20% advantage in efficiency, they could end up with a 10 to 100 fold penalty to power density. In other words, for the same mass budget, a spaceship is likely to output much less beam power when using an XFEL than when compared to using a particle beam.
X-rays are very hard to manipulate. There exists no mirror that can reflect them and no lens which can focus them.
An XFEL would have to rely on grazing-incidence optics to aim and focus its beam. Grazing-incidence optics are nestled cones of a dense metal like gold angled at 1 degree or less.
Such an optic would need many, many such cones even at small sizes. As the focal distance increases, the angle of the cones must decrease. A 1 meter diameter optic focused at a spot 10,000km away requires cones of an angle 2.86 *10^-6 degrees. This leads to optics composed of no less than millions of cones, which is incredibly impractical and necessarily heavy. It should also be noted that grazing incidence optics cannot adjust their focal point.
The alternative is a Fresnel Zone plate. It is not as delicate or as intricate as a grazing incidence optic, can adjust its focus and can be a rather lightweight device even when several meters wide. The ‘catch’ is that it absorbs between 50 and 75% of the laser beam.
The plate also needs to be actively cooled and reduces the efficiency of an XFEL far below that of a particle beam accelerator.
Does this mean particle beams are safe? Not yet!

XFELs might regain the upper hand by using new methods of focusing X-rays.
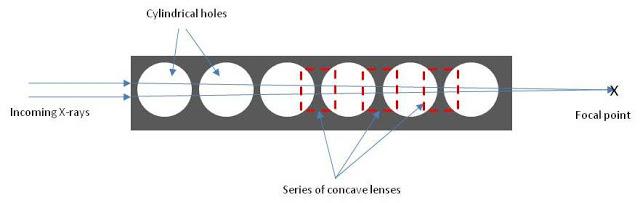
Bent diffraction crystals can reach high diffraction efficiency, which would make them very good at focusing X-rays. There could be further development of highly efficient Kinoform lenses.
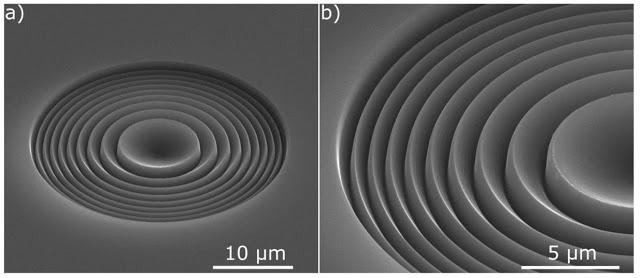
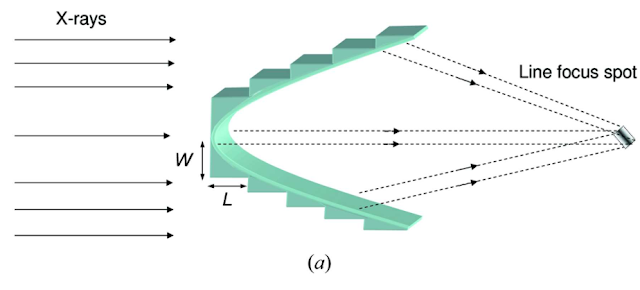
Alternatively, XFELs enjoying improvements in accelerator technology and undulator field strength can produce extremely short wavelengths, lower than 0.0001 nanometers, without requiring structures of several hundreds of meters in length. No focusing optics are needed in this case! Even a millimeter wide opening from an undulator is enough to produce beams of 0.3 nanoradian divergence. This performance is no more than 10x what is achievable today.
XFELs might regain the upper hand by using new methods of focusing X-rays.
Bent diffraction crystals can reach high diffraction efficiency, which would make them very good at focusing X-rays. There could be further development of highly efficient Kinoform lenses.
Alternatively, XFELs enjoying improvements in accelerator technology and undulator field strength can produce extremely short wavelengths, lower than 0.0001 nanometers, without requiring structures of several hundreds of meters in length. No focusing optics are needed in this case! Even a millimeter wide opening from an undulator is enough to produce beams of 0.3 nanoradian divergence. This performance is no more than 10x what is achievable today.
We could therefore expect XFELs to end up creating 60 cm spot sizes at million kilometre distances. Their only limitation would be light lag. Can particle beams be competitive with such lasers?
Cold Ions
The most effective way to reduce the divergence of a particle beam down to its neutralization-imposed minimum is to reduce the emittance of the ion source.
The ions released from an ion source usually have a ‘temperature’ of about 1 eV. We have assumed so far that the particle accelerator uses the ions at this temperature and does not significantly increase or decrease it. This does not have to be the case.
Ions can be cooled after exiting their source and before they are accelerated to higher energies. Beam cooling techniques that can be used here include stochastic, radiative and electron cooling.
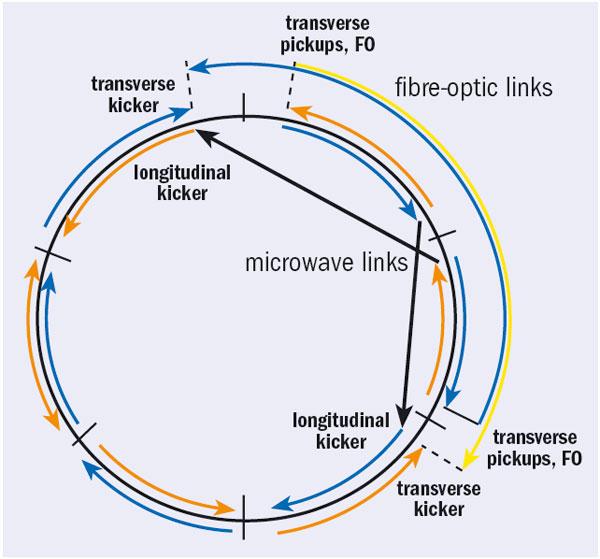
Stochastic cooling inside a low-energy ring uses electromagnets to try to correct the path of a beam so that all the particles have a very similar temperature instead of a range of temperatures.
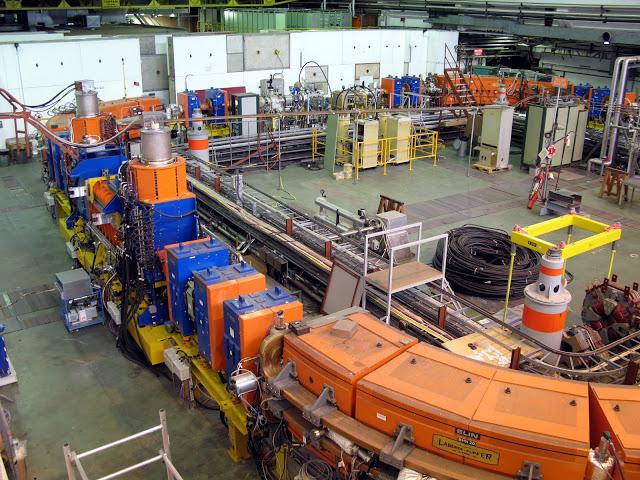
Radiative cooling only works practically with electron beams. It forces the electrons to wiggle to maximize their energy loss through Bremsstrahlung radiation. Energy is then added back to the electrons only in the longitudinal direction, leading to a gradual reduction in transverse temperature.
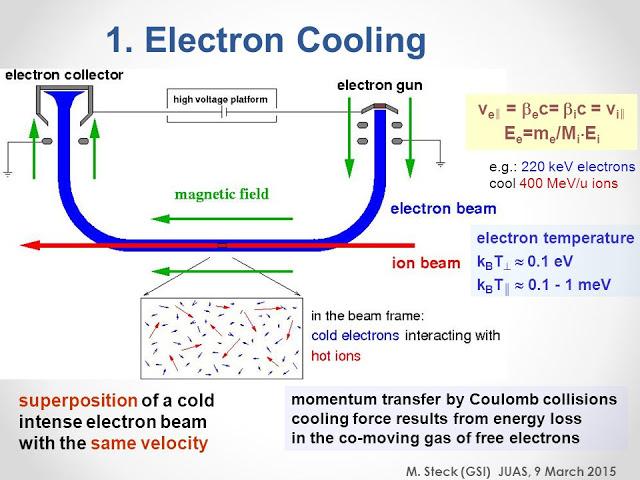
Electron cooling involves mixing hot ions with cold electrons (themselves cooled radiatively) to reduce the ion temperature. After mixing, the beam is bent by electromagnets so that the ions and electrons separate into different paths.
All these techniques require multiple passes to be truly effective and therefore work best in a ring-shaped accelerator. Low energy ions produced by an ion source are easily bent into a circle by lightweight magnets. Over the course of several seconds or even minutes, ions are run around and around the the ring and passed multiple times through a cooling section. Eventually, the ion temperature approaches that of the electrons.
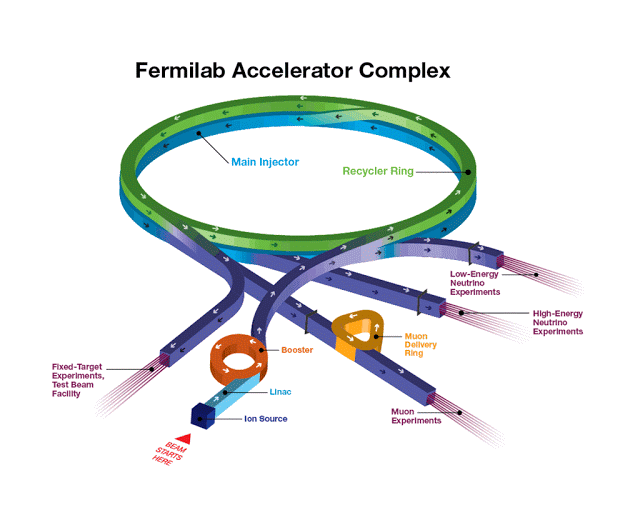
Regular electrons will have the temperature of their emitter, 2000 to 3000 K, which corresponds to 0.17 to 0.26 eV. A photocathode emitter, where laser light is used to expel electrons from a surface, can produce electrons as cool as 1.5 meV or 17.4 K. They will be further cooled down to the tens of kelvins.
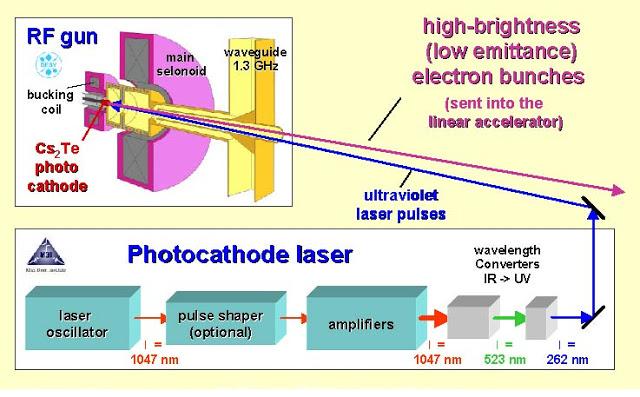
If such low electron temperatures are accessible, and a ring accelerator can be used to bring ions down to similar temperatures, then there is the possibility of of creating cryogenic ion beams. They would have a near-zero emittance if it were not for electrostatic repulsion and internal collisions between the charged particles.
Cold Ions
The most effective way to reduce the divergence of a particle beam down to its neutralization-imposed minimum is to reduce the emittance of the ion source.
The ions released from an ion source usually have a ‘temperature’ of about 1 eV. We have assumed so far that the particle accelerator uses the ions at this temperature and does not significantly increase or decrease it. This does not have to be the case.
Ions can be cooled after exiting their source and before they are accelerated to higher energies. Beam cooling techniques that can be used here include stochastic, radiative and electron cooling.
Stochastic cooling inside a low-energy ring uses electromagnets to try to correct the path of a beam so that all the particles have a very similar temperature instead of a range of temperatures.
Radiative cooling only works practically with electron beams. It forces the electrons to wiggle to maximize their energy loss through Bremsstrahlung radiation. Energy is then added back to the electrons only in the longitudinal direction, leading to a gradual reduction in transverse temperature.
Electron cooling involves mixing hot ions with cold electrons (themselves cooled radiatively) to reduce the ion temperature. After mixing, the beam is bent by electromagnets so that the ions and electrons separate into different paths.
All these techniques require multiple passes to be truly effective and therefore work best in a ring-shaped accelerator. Low energy ions produced by an ion source are easily bent into a circle by lightweight magnets. Over the course of several seconds or even minutes, ions are run around and around the the ring and passed multiple times through a cooling section. Eventually, the ion temperature approaches that of the electrons.
Regular electrons will have the temperature of their emitter, 2000 to 3000 K, which corresponds to 0.17 to 0.26 eV. A photocathode emitter, where laser light is used to expel electrons from a surface, can produce electrons as cool as 1.5 meV or 17.4 K. They will be further cooled down to the tens of kelvins.
If such low electron temperatures are accessible, and a ring accelerator can be used to bring ions down to similar temperatures, then there is the possibility of of creating cryogenic ion beams. They would have a near-zero emittance if it were not for electrostatic repulsion and internal collisions between the charged particles.
Cryogenic particle beams are the solution to obtaining divergence no greater than the minimum imposed by neutralization disturbance, and this does not involve any expanding or focusing optics.
There is a secondary advantage to cryogenic particle beams that can allow them to reach divergence even lower than the neutralization minimum.
A bunch of neutral atoms travelling through space in a dense stream is not really different than a quick-moving gas. Cold gases condense.
Cold hydrogen atoms condense into H2 droplets. Nitrogen condenses into N2. Cesium atoms condense into solid metal balls. The higher the vaporization temperature (hydrogen boils at 10 K in vacuum, but nitrogen only needs to be cooler than 63 K), the more likely that the particle beam condenses into a stream of higher molar mass entities.
When two atoms join into a molecule, they average their transverse velocities. This effectively reduces the divergence of a particle beam by 42%.

The square root of the mass ratio between the final condensed product and the individual atoms gives the reduction in divergence. This phenomenon was critical to Geoffrey A. Landis’s ‘Interstellar Flight by Particle Beam’ paper, where he suggested that mercury atoms would condense into million-particle droplets.
There is a secondary advantage to cryogenic particle beams that can allow them to reach divergence even lower than the neutralization minimum.
A bunch of neutral atoms travelling through space in a dense stream is not really different than a quick-moving gas. Cold gases condense.
Cold hydrogen atoms condense into H2 droplets. Nitrogen condenses into N2. Cesium atoms condense into solid metal balls. The higher the vaporization temperature (hydrogen boils at 10 K in vacuum, but nitrogen only needs to be cooler than 63 K), the more likely that the particle beam condenses into a stream of higher molar mass entities.
When two atoms join into a molecule, they average their transverse velocities. This effectively reduces the divergence of a particle beam by 42%.
The square root of the mass ratio between the final condensed product and the individual atoms gives the reduction in divergence. This phenomenon was critical to Geoffrey A. Landis’s ‘Interstellar Flight by Particle Beam’ paper, where he suggested that mercury atoms would condense into million-particle droplets.
With ion cooling and condensation, we can obtain the sub-nanoradian particle beams necessary to compete with future XFEL performance.
Going further, we could expect beams of multiple species. Carbon and hydrogen beam are especially interesting. Carbon atoms at 1000 MeV could be released in parallel with hydrogen atoms at 80.3 MeV. Some of the hydrogen condenses into H2 and eventually diverges away, but they only carry a fraction of the energy. Carbon and hydrogen could instead condense into methane. The divergence of the carbon beam would be reduced by a 13.4%, and that of the hydrogen beam by 50%. When the methane molecules strike a target surface, they separate back into carbon and hydrogen. The carbon would stop very quickly and deposit all of its energy near the surface. The hydrogen is much more penetrating and would continue deeper into the material.
A methane beam would deliver both thermal and radiation damage and help defeat protection optimized for one or the other, all while maintaining a divergence much smaller than a pure radiation beam. Even better, the carbon would help ‘carry’ penetrating hydrogen particles through magnetic fields that would otherwise deflect lightweight hydrogen on its own. Methane is after all just 8% easier to ionize than hydrogen but 16 times harder to deflect.
Laser Coupled Particle Beams
Going further, we could expect beams of multiple species. Carbon and hydrogen beam are especially interesting. Carbon atoms at 1000 MeV could be released in parallel with hydrogen atoms at 80.3 MeV. Some of the hydrogen condenses into H2 and eventually diverges away, but they only carry a fraction of the energy. Carbon and hydrogen could instead condense into methane. The divergence of the carbon beam would be reduced by a 13.4%, and that of the hydrogen beam by 50%. When the methane molecules strike a target surface, they separate back into carbon and hydrogen. The carbon would stop very quickly and deposit all of its energy near the surface. The hydrogen is much more penetrating and would continue deeper into the material.
A methane beam would deliver both thermal and radiation damage and help defeat protection optimized for one or the other, all while maintaining a divergence much smaller than a pure radiation beam. Even better, the carbon would help ‘carry’ penetrating hydrogen particles through magnetic fields that would otherwise deflect lightweight hydrogen on its own. Methane is after all just 8% easier to ionize than hydrogen but 16 times harder to deflect.
Laser Coupled Particle Beams
There is another method of reducing emittance which is radically different from those mentioned so far. It is based on optical tweezers that are known for trapping and manipulating tiny particles using laser beams.
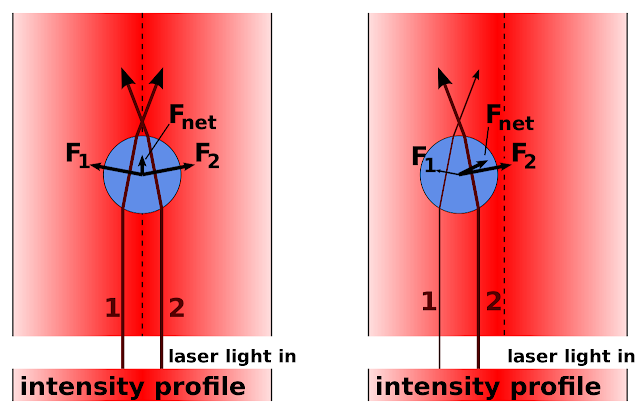
The same method can be used to control the motion of particles in a particle beam. It is studied as part of NASA’s Innovative Advanced Concepts, promising diffractionless beams across interstellar distances. We will call them ‘Laser-Couple Particle Beams’ or LCPBs.

Can be applied in the context of distances on the order of 1,000 to 1,000,000 kilometers?
A laser beam can be used to create a column of light pointed at a target. The intensity of the laser varies from the edge to the center of the column in a gaussian profile. To simplify, we can say that the laser at the center of the column is twice as intense as the average intensity.
When a particle beam is inserted into the column and travels parallel to it, it experiences a force pushing its particles towards the center of the column. That is the gradient force.

We are solely interested in particles sitting at the edge of the column, as they determine the divergence. They experience the full pull of the gradient force.
As best that can be determined, the maximal acceleration towards the center of the laser column that a particle experiences is as follows:
AP is the atomic polarizability.
IG is the intensity gradient of the laser, in W/m^2/m
AAG is the average acceleration factor, drawn from the table below.
PM is the particle mass in kg.
The AAG is the result of the particles experiencing less and less gradient force drawing them towards the center of the laser column as they come closer. It is the result of the intensity gradient falling as they do so. The greater the reduction in divergence we want, the lower the average acceleration the particles experience.
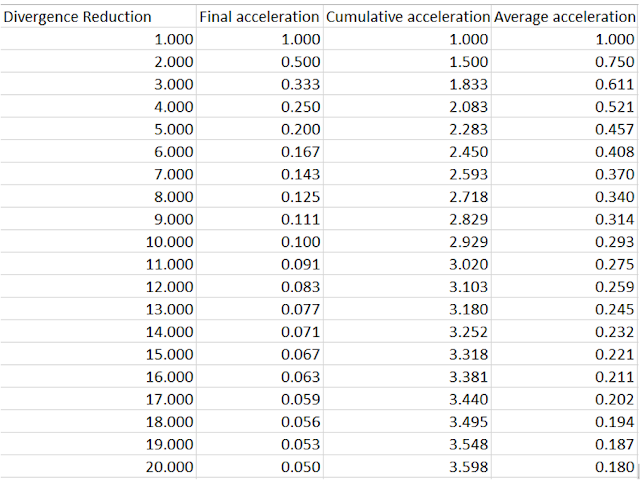
From the table, we can read that if we want to reduce the divergence by a factor 8, the average acceleration the particles experience is 34% of the initial acceleration.
The same method can be used to control the motion of particles in a particle beam. It is studied as part of NASA’s Innovative Advanced Concepts, promising diffractionless beams across interstellar distances. We will call them ‘Laser-Couple Particle Beams’ or LCPBs.
Can be applied in the context of distances on the order of 1,000 to 1,000,000 kilometers?
A laser beam can be used to create a column of light pointed at a target. The intensity of the laser varies from the edge to the center of the column in a gaussian profile. To simplify, we can say that the laser at the center of the column is twice as intense as the average intensity.
When a particle beam is inserted into the column and travels parallel to it, it experiences a force pushing its particles towards the center of the column. That is the gradient force.
We are solely interested in particles sitting at the edge of the column, as they determine the divergence. They experience the full pull of the gradient force.
As best that can be determined, the maximal acceleration towards the center of the laser column that a particle experiences is as follows:
- Average Particle Acceleration = (0.5 * AP * IG * DRF)/PM
AP is the atomic polarizability.
IG is the intensity gradient of the laser, in W/m^2/m
AAG is the average acceleration factor, drawn from the table below.
PM is the particle mass in kg.
The AAG is the result of the particles experiencing less and less gradient force drawing them towards the center of the laser column as they come closer. It is the result of the intensity gradient falling as they do so. The greater the reduction in divergence we want, the lower the average acceleration the particles experience.
From the table, we can read that if we want to reduce the divergence by a factor 8, the average acceleration the particles experience is 34% of the initial acceleration.
AP values can be found here (https://cccbdb.nist.gov/pollistx.asp) in multiples of the radius cubed.
Hydrogen, with a radius of 5.29 * 10^-11 m, would have a polarizability of 0.667 * (2.5 * 10^-11)^3: 1.04*10^-32.
Potassium, with a radius of 2.2*10^-10 m, would have a polarizability of 43 * (2.2*10^-10)^3: 4.58*10^-28.
IG for a gaussian beam is roughly twice the average laser intensity (W/m^2) divided by the laser beam’s radius.
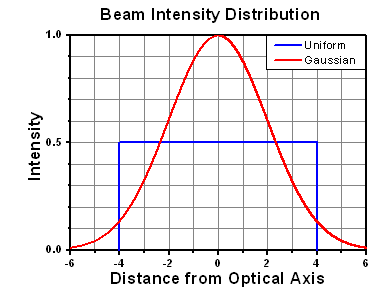
Using three worked examples, we can clearly see that LCPBs can have massively extended ranges when compared to either lasers or particle beams alone. We assume that they have received the beam cooling treatment and that their initial divergence is roughly equal to the minimum imposed by neutralization.
In the first example, we will calculate the laser parameters needed to reduce the divergence of a 1 meter wide, 100 MeV hydrogen beam from 54 nanoradians to 5.4 nanoradians within a distance of 1,000 km.
Hydrogen at 100 MeV is travelling at 0.428 C or 128,360 km/s, so it crosses a distance of 1,000 km in about 7.79 milliseconds. An initial divergence of 54 nanoradians implies that it spreads at a rate of 6.93 m/s. We therefore need to reduce the transverse velocity from 6.93 m/s to 0.69 m/s within 7.79 milliseconds. That is an acceleration of 801 m/s^2.
We’re trying to reduce the divergence by a factor 10, so the average acceleration is 29.3% of the initial acceleration. Conversely, the initial acceleration will have to be 3.41 times greater to achieve the desired average acceleration, about 2732 m/s^2.
We input PA = 2732 m/s^2, AP = 1.04*10^-32 and PM = 1.67*10^-27 kg. From that, we determine that IG = 8.8*10^8 W/m^2/m
Since the beam is 1 meter in diameter, it has a radius of 0.5 meters, so average laser intensity will be 219.4 MW/m^2. This is not unreasonable. The total laser power required is 172.3 MW. It is quite a big penalty to the power requirements of the particle beam.
In the second example, we will calculate the laser parameters needed to reduce the divergence of a 1 meter wide, 300 MeV Xenon beam from 2.5 nanoradians to 0.25 nanoradians within a distance of 1,000 km.
Xenon at 300 MeV is travelling at 0.0697 C or 20,890 km/s, so it crosses a distance of 1,000 km in about 47.87 milliseconds. An initial divergence of 2.5 nanoradians implies that it spreads at a rate of 0.05 m/s. We therefore need to reduce the transverse velocity from 0.05 m/s to 0.005 m/s within 47.87 milliseconds. That is an acceleration of 0.982 m/s^2.
The divergence reduction factor is 10, so the average acceleration is
29.3% of the initial acceleration. We therefore need an initial acceleration of 3.34 m/s^2.
We input PA = 3.34 m/s^2, AP = 5.04*10^-30 and PM = 2.18*10^-25 kg. From that, we determine that IG = 2.89*10^5 W/m^2/m
The average laser intensity will be 72.4 kW/m^2 and total laser power is just 56.7 kW! This is a very minor penalty to a particle beam accelerator with a several megawatt output.
In the final example, let’s try for a very narrow beam, using condensation to produce a molecule with very low initial divergence and high polarizability. Lets’ also be generous with the particle energy.
Starting with 1000 MeV Cesium (min divergence 0.43 nrad) and 955 MeV Iodine (min divergence 1 nrad), we expect to end up with Cesium Iodide with a polarizability of 1.94*10^-28, based on an average of the two element’s Van der Wall’s radii figures from here. The divergence of the molecule is 0.7 nrad and it will be travelling at 0.126 C or 37,800 km/s.
What laser is needed to reduce the divergence of a 1 millimeter wide, Cesium-Iodine beam from 0.7 nanoradians to 0.035 nanoradians within a distance of 1,000 km?
The 0.126 C beam crosses a distance of 1,000 km in about 26.45 milliseconds. An initial divergence of 0.7 nanoradians implies that it spreads at a rate of 0.021 m/s. We therefore need to reduce the transverse velocity from 0.021 m/s to 0.00108 m/s within 26.45 milliseconds. That is an acceleration of 0.776 m/s^2.
The divergence reduction factor is 20, so the average acceleration is
18% of the initial acceleration. We therefore need an initial acceleration of 4.3 m/s^2.
We input PA = 4.3 m/s^2, AP = 1.94*10^-28 and PM = 4.32*10^-25 kg. From that, we determine that IG = 1.92*10^4 W/m^2/m
The average laser intensity will be 4.78 W/m^2 so total laser power is a rather incredible 0.37 … milliWatts.
Summary of results:
Example 1
Particle: 100 MeV Hydrogen
Initial divergence: 54 nrad
Final divergence: 5.4 nrad
Power required: 172.3 MW
Potassium, with a radius of 2.2*10^-10 m, would have a polarizability of 43 * (2.2*10^-10)^3: 4.58*10^-28.
IG for a gaussian beam is roughly twice the average laser intensity (W/m^2) divided by the laser beam’s radius.
Using three worked examples, we can clearly see that LCPBs can have massively extended ranges when compared to either lasers or particle beams alone. We assume that they have received the beam cooling treatment and that their initial divergence is roughly equal to the minimum imposed by neutralization.
In the first example, we will calculate the laser parameters needed to reduce the divergence of a 1 meter wide, 100 MeV hydrogen beam from 54 nanoradians to 5.4 nanoradians within a distance of 1,000 km.
Hydrogen at 100 MeV is travelling at 0.428 C or 128,360 km/s, so it crosses a distance of 1,000 km in about 7.79 milliseconds. An initial divergence of 54 nanoradians implies that it spreads at a rate of 6.93 m/s. We therefore need to reduce the transverse velocity from 6.93 m/s to 0.69 m/s within 7.79 milliseconds. That is an acceleration of 801 m/s^2.
We’re trying to reduce the divergence by a factor 10, so the average acceleration is 29.3% of the initial acceleration. Conversely, the initial acceleration will have to be 3.41 times greater to achieve the desired average acceleration, about 2732 m/s^2.
We input PA = 2732 m/s^2, AP = 1.04*10^-32 and PM = 1.67*10^-27 kg. From that, we determine that IG = 8.8*10^8 W/m^2/m
Since the beam is 1 meter in diameter, it has a radius of 0.5 meters, so average laser intensity will be 219.4 MW/m^2. This is not unreasonable. The total laser power required is 172.3 MW. It is quite a big penalty to the power requirements of the particle beam.
In the second example, we will calculate the laser parameters needed to reduce the divergence of a 1 meter wide, 300 MeV Xenon beam from 2.5 nanoradians to 0.25 nanoradians within a distance of 1,000 km.
Xenon at 300 MeV is travelling at 0.0697 C or 20,890 km/s, so it crosses a distance of 1,000 km in about 47.87 milliseconds. An initial divergence of 2.5 nanoradians implies that it spreads at a rate of 0.05 m/s. We therefore need to reduce the transverse velocity from 0.05 m/s to 0.005 m/s within 47.87 milliseconds. That is an acceleration of 0.982 m/s^2.
The divergence reduction factor is 10, so the average acceleration is
29.3% of the initial acceleration. We therefore need an initial acceleration of 3.34 m/s^2.
We input PA = 3.34 m/s^2, AP = 5.04*10^-30 and PM = 2.18*10^-25 kg. From that, we determine that IG = 2.89*10^5 W/m^2/m
The average laser intensity will be 72.4 kW/m^2 and total laser power is just 56.7 kW! This is a very minor penalty to a particle beam accelerator with a several megawatt output.
In the final example, let’s try for a very narrow beam, using condensation to produce a molecule with very low initial divergence and high polarizability. Lets’ also be generous with the particle energy.
Starting with 1000 MeV Cesium (min divergence 0.43 nrad) and 955 MeV Iodine (min divergence 1 nrad), we expect to end up with Cesium Iodide with a polarizability of 1.94*10^-28, based on an average of the two element’s Van der Wall’s radii figures from here. The divergence of the molecule is 0.7 nrad and it will be travelling at 0.126 C or 37,800 km/s.
What laser is needed to reduce the divergence of a 1 millimeter wide, Cesium-Iodine beam from 0.7 nanoradians to 0.035 nanoradians within a distance of 1,000 km?
The 0.126 C beam crosses a distance of 1,000 km in about 26.45 milliseconds. An initial divergence of 0.7 nanoradians implies that it spreads at a rate of 0.021 m/s. We therefore need to reduce the transverse velocity from 0.021 m/s to 0.00108 m/s within 26.45 milliseconds. That is an acceleration of 0.776 m/s^2.
The divergence reduction factor is 20, so the average acceleration is
18% of the initial acceleration. We therefore need an initial acceleration of 4.3 m/s^2.
We input PA = 4.3 m/s^2, AP = 1.94*10^-28 and PM = 4.32*10^-25 kg. From that, we determine that IG = 1.92*10^4 W/m^2/m
The average laser intensity will be 4.78 W/m^2 so total laser power is a rather incredible 0.37 … milliWatts.
Summary of results:
Example 1
Particle: 100 MeV Hydrogen
Initial divergence: 54 nrad
Final divergence: 5.4 nrad
Power required: 172.3 MW
Example 2
Particle: 300 MeV Xenon
Initial divergence: 2.5 nrad
Final divergence: 0.25 nrad
Power required: 56.7 kW
Particle: 300 MeV Xenon
Initial divergence: 2.5 nrad
Final divergence: 0.25 nrad
Power required: 56.7 kW
Example 3
Particle: 1000 MeV Cesium and 955 MeV Iodine
Initial divergence: 0.7 nrad
Final divergence: 0.035 nrad
Power required: 0.37 mW
These are the theoretical minimum values, assuming maximal optical tweezer gradient force and that 100% of the laser energy goes towards pushing particles together. However, if even a fraction of this performance is attainable (you can always compensate with stronger lasers, rising from milliwatts to watts), then particle beams with a tenth of a nanoradian in divergence are attainable.
In the final example, we find that LCPBs are able to act like ‘pencil beams’ that barely spread from the moment they exit their millimeter-sized ports to when they produce spot sizes on the order of 3.5 cm at 1 million km.
Fighting with LCPBs
It should be evident by now that if we start off with a beam of low divergence and narrow diameter, we can use very moderate laser power to significantly reduce divergence. LCPB ‘pencil beams’ would be able to produce small spot sizes at extreme distances. They would dominate the field of directed energy weapons and would be the ideal power transmission tool for interplanetary or interstellar propulsion. A 0.1 nanoradian divergence beam would concentrate its energy within a 0.03 m^2 spot at a million kilometers, or catch a 100 meter wide receiver from a distance of 500 million km. The latter is 33% more than the distance from the Earth to Mars… if they were on opposite sides of the Sun!
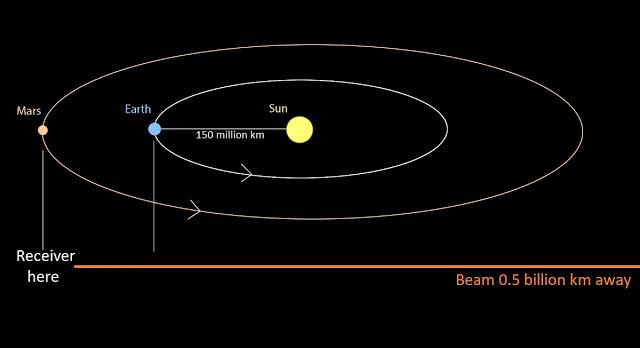
LCPBs will dominate only within distances that they can reliably hit a target though. Their main limitation would be their velocity. The beam velocity does not matter when aiming at something that does not move appreciably in the time it takes the beam to reach it, or has a well defined trajectory, like an asteroid or a space station. LCPBs suffer instead when aiming at randomly maneuvering targets. The same random ‘jinking’ used to avoid lasers at distances where light lag is significant can be used at shorter ranges against the slower particle beams.
Particle: 1000 MeV Cesium and 955 MeV Iodine
Initial divergence: 0.7 nrad
Final divergence: 0.035 nrad
Power required: 0.37 mW
These are the theoretical minimum values, assuming maximal optical tweezer gradient force and that 100% of the laser energy goes towards pushing particles together. However, if even a fraction of this performance is attainable (you can always compensate with stronger lasers, rising from milliwatts to watts), then particle beams with a tenth of a nanoradian in divergence are attainable.
In the final example, we find that LCPBs are able to act like ‘pencil beams’ that barely spread from the moment they exit their millimeter-sized ports to when they produce spot sizes on the order of 3.5 cm at 1 million km.
Fighting with LCPBs
It should be evident by now that if we start off with a beam of low divergence and narrow diameter, we can use very moderate laser power to significantly reduce divergence. LCPB ‘pencil beams’ would be able to produce small spot sizes at extreme distances. They would dominate the field of directed energy weapons and would be the ideal power transmission tool for interplanetary or interstellar propulsion. A 0.1 nanoradian divergence beam would concentrate its energy within a 0.03 m^2 spot at a million kilometers, or catch a 100 meter wide receiver from a distance of 500 million km. The latter is 33% more than the distance from the Earth to Mars… if they were on opposite sides of the Sun!
LCPBs will dominate only within distances that they can reliably hit a target though. Their main limitation would be their velocity. The beam velocity does not matter when aiming at something that does not move appreciably in the time it takes the beam to reach it, or has a well defined trajectory, like an asteroid or a space station. LCPBs suffer instead when aiming at randomly maneuvering targets. The same random ‘jinking’ used to avoid lasers at distances where light lag is significant can be used at shorter ranges against the slower particle beams.
Let’s take an enemy warship with a hull 10 meters wide. It can accelerate sideways at 1m/s^2.
If the beam travels at 0.07 C, like in the second example, then it will only hit that warship with 100% certainty out to a distance of 66,000 km. Accepting a hit rate of 50% only increases the distance to 77,000 km. It is a great distance, but only a tiny fraction of what the LCPB is capable of.
The enemy warship can reduce these ranges by accelerating harder. At 10m/s^2, the 100% hit distance is ‘just’ 21,000km. Of course, this consumes ten times more propellant.
A dumb but surefire way to defeat this enemy warship is to keep firing the LCPB until the jinking has consumed of its deltaV and renders it a non-maneuvering target. This might take minutes to days depending on the capabilities of the propulsion technology, and can be greatly extended by moving further away and reducing the acceleration. During this period, the enemy is firing back, a unenviable situation.
Smarter heads would choose to fire along a pattern that touches upon all potential positions the enemy warship can take. This way, a low hit chance can be converted into a low hit rate.
For example, a 0.07 C beam targeting a 10m wide target with 1 m/s^2 of acceleration would only have a hit chance of 1/82 at a distance of 200,000 km.
Instead of shooting spots at a random within the area of probable positions the target can take, the area is divided into sections, with each section given a fraction of the beam’s output. This way, instead of doing full damage with a 1.22% chance, we deal 1.22% damage all the time.
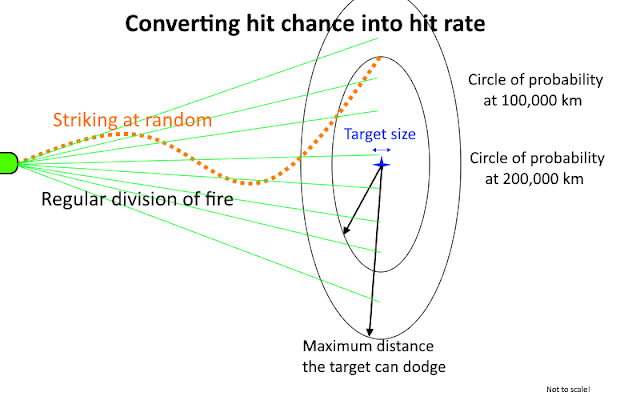
A 1000 MW LCPB with a 0.1 nanoradian divergence would have a spot size of 4 cm at 200,000 km. It should be able to burn through 24.6 m/s of graphite or 9.72 m/s of steel against an immobile target. Even after being divided and spread into 82 section to ensure a 100% hit chance, this is enough to maintain an average penetration rate of 30 cm/s against graphite and 11.8 cm/s against steel.
If the beam travels at 0.07 C, like in the second example, then it will only hit that warship with 100% certainty out to a distance of 66,000 km. Accepting a hit rate of 50% only increases the distance to 77,000 km. It is a great distance, but only a tiny fraction of what the LCPB is capable of.
The enemy warship can reduce these ranges by accelerating harder. At 10m/s^2, the 100% hit distance is ‘just’ 21,000km. Of course, this consumes ten times more propellant.
A dumb but surefire way to defeat this enemy warship is to keep firing the LCPB until the jinking has consumed of its deltaV and renders it a non-maneuvering target. This might take minutes to days depending on the capabilities of the propulsion technology, and can be greatly extended by moving further away and reducing the acceleration. During this period, the enemy is firing back, a unenviable situation.
Smarter heads would choose to fire along a pattern that touches upon all potential positions the enemy warship can take. This way, a low hit chance can be converted into a low hit rate.
For example, a 0.07 C beam targeting a 10m wide target with 1 m/s^2 of acceleration would only have a hit chance of 1/82 at a distance of 200,000 km.
Instead of shooting spots at a random within the area of probable positions the target can take, the area is divided into sections, with each section given a fraction of the beam’s output. This way, instead of doing full damage with a 1.22% chance, we deal 1.22% damage all the time.
A 1000 MW LCPB with a 0.1 nanoradian divergence would have a spot size of 4 cm at 200,000 km. It should be able to burn through 24.6 m/s of graphite or 9.72 m/s of steel against an immobile target. Even after being divided and spread into 82 section to ensure a 100% hit chance, this is enough to maintain an average penetration rate of 30 cm/s against graphite and 11.8 cm/s against steel.
Closing the distance greatly improves these numbers, as the beam becomes more intense and the target can take up less positions, so the beam power doesn’t have to be divided as much.
Performance table for a 1000 MW 0.1 nanoradian beam vs 10m target:
Base penetration rates
Target Material/Distance: Penetration rate
Graphite / 500,000 km: 0.843 m/s
Graphite / 250,000 km: 3.36 m/s
Graphite / 100,000 km: 20.8 m/s
Steel / 500,000 km: 1.76 m/s
Steel / 250,000 km: 6.46 m/s
Steel / 100,000 km: 32.9 m/s
Beam velocity: 0.01 C
Target acceleration/Distance/Sections: Average penetration rate vs graphite
Performance table for a 1000 MW 0.1 nanoradian beam vs 10m target:
Base penetration rates
Target Material/Distance: Penetration rate
Graphite / 500,000 km: 0.843 m/s
Graphite / 250,000 km: 3.36 m/s
Graphite / 100,000 km: 20.8 m/s
Steel / 500,000 km: 1.76 m/s
Steel / 250,000 km: 6.46 m/s
Steel / 100,000 km: 32.9 m/s
Beam velocity: 0.01 C
Target acceleration/Distance/Sections: Average penetration rate vs graphite
1m/s^2 / 500,000km / 7,716,050: 0.11 micrometers/s
1m/s^2 / 250,000km / 482,250: 7 micrometers/s
1m/s^2 / 100,000km / 12,345: 1.7 millimeters/s
10m/s^2 / 250,000km / 48,225,300: 0.07 micrometers/s
10m/s^2 / 100,000km / 1,234,570: 16.8 micrometers/s
Beam velocity: 0.1 C
Target acceleration/Distance/Sections: Average penetration rate vs graphite
1m/s^2 / 500,000km / 771: 1.1 mm/s
1m/s^2 / 250,000km / 48: 70 mm/s
1m/s^2 / 100,000km / 1: 20.8 m/s
10m/s^2 / 250,000km / 4,822: 0.7 mm/s
10m/s^2 / 100,000km / 123: 169 mm/s
Beam velocity: 0.5 C
Target acceleration/Distance/Sections: Average penetration rate vs graphite
1m/s^2 / 500,000km / 1: 0.843 m/s
1m/s^2 / 250,000km / 1: 3.36 m/s
1m/s^2 / 100,000km / 1: 20.8 m/s
10m/s^2 / 250,000km / 8: 0.42 m/s
10m/s^2 / 100,000km / 1: 20.8 m/s
Based on these findings, LCPBs for combat would best be designed around produce a beam balanced between having enough speed to catch maneuvering targets and enough weight (molar mass) to maintain a low divergence.
Against large, relatively immobile targets like a space station or an asteroid fort, it is very easy to create very slow and very heavy beams that maintain an extremely low divergence across interplanetary distances.
Such ‘anti-fort’ beams would have penetration rates that could poke holes through hundreds of meters of rock and steel. The huge mass that serves as a great heatsink and helps ward off smaller warships using weapons of greater power and thus range in a laser vs laser scenario becomes a hindrance in an LCPB vs LCPB scenario.
The asteroid forts could try to retaliate with their own beams, but they will not be able to hurt maneuvering targets (look at how much average penetration drops as the number of sections increases) using LCPBs nor match their range using lasers.
Therefore, we can safely say that any large, mostly immobile structure can be defeated by a maneuvering LCPB-equipped spaceship.
The situation is more nuanced when it is a maneuvering spaceship against a Laser Weapon Web. LCPBs will lose if the LWW is able to bring their ‘terminal mirrors’ close to the LCPB to match its range faster than the LCPB or a radiation weapon can destroy them. This assumes the battles does not start with the LCPB-equipped spaceship already in range of a Laser Weapon Web, and if the power source of the LWW isn’t a planet protected by a thick atmosphere that particle beams cannot get through…
For SF authors and game designers, LCPBs have interesting tactical consequences.
The name of the game is balance.
The low-risk strategy in a one vs one situation is to spread the beam’s output and wait for the armor to be whittled away. A high-risk alternative is to drag the beam at random around the target and hope that when the beam does connect, it’s full-power penetration rate is enough to cut through the armor layer and get at the vital internal components. With computers and predictive software, a middle ground is likely to be sought instead: the target’s motions are predicted to lie along a certain path, and it is better to try to focus on getting a few good hits that get through the armor to start reducing the target’s ability to maneuver or fire back.
A balanced strategy with an optimum level of armor would deal damage the quickest and avoid harm the longest. Having massively more powerful beams, thicker armor or faster acceleration will not gain any decisive victory.
That is only passive armor though. We must include the threat from radiation beams and the option of active defenses.
Radiation beams would easily pass through the ‘optimal armor’ from an LCPB-balanced design. They require powerful magnetic fields to deflect instead. However, the power requirements and weight of equipment to surround a spaceship with a magnetic field sufficient to deflect the beam from an accelerator a fraction of the size and weight quickly becomes untenable. Instead of increasing the field strength to match the bending radius of more and more energetic particles, it is possible instead of physically move a smaller field outwards and into the beam path.
Smaller magnetic field generators can be fitted on drones and placed a kilometer or so away from the main spaceship. The ‘mag-shield’ drones only need to be strong enough to cause the incoming radiation to bend by an angle sufficient to miss the main spaceship.
For example, protecting a 10 meter wide spaceship using a mag-shield drone 1000 meters in front of it only requires that incoming beams be deflected by 0.29 degrees! This naturally allows for very small and weak fields to be used.
The downside to mag-shield drones is that they only work against beams coming from a small number of directions. An unexpected attack would be devastating. It wouldn’t have to be very powerful or intense to severely harm a living crew or sensitive electrons, as was demonstrated in the previous Particle Beam post. In fact, it could be the ideal weapon for deployment by cryogenic stealth craft, as they are the ones which could sneak into flanking attack vector and the low power requirements of a radiation weapon allows its waste heat to be entirely absorbed by the on-board cryogenic heatsink for minutes or even hours at a time.
That is only passive armor though. We must include the threat from radiation beams and the option of active defenses.
Radiation beams would easily pass through the ‘optimal armor’ from an LCPB-balanced design. They require powerful magnetic fields to deflect instead. However, the power requirements and weight of equipment to surround a spaceship with a magnetic field sufficient to deflect the beam from an accelerator a fraction of the size and weight quickly becomes untenable. Instead of increasing the field strength to match the bending radius of more and more energetic particles, it is possible instead of physically move a smaller field outwards and into the beam path.
Smaller magnetic field generators can be fitted on drones and placed a kilometer or so away from the main spaceship. The ‘mag-shield’ drones only need to be strong enough to cause the incoming radiation to bend by an angle sufficient to miss the main spaceship.
For example, protecting a 10 meter wide spaceship using a mag-shield drone 1000 meters in front of it only requires that incoming beams be deflected by 0.29 degrees! This naturally allows for very small and weak fields to be used.
The downside to mag-shield drones is that they only work against beams coming from a small number of directions. An unexpected attack would be devastating. It wouldn’t have to be very powerful or intense to severely harm a living crew or sensitive electrons, as was demonstrated in the previous Particle Beam post. In fact, it could be the ideal weapon for deployment by cryogenic stealth craft, as they are the ones which could sneak into flanking attack vector and the low power requirements of a radiation weapon allows its waste heat to be entirely absorbed by the on-board cryogenic heatsink for minutes or even hours at a time.
A second line of defense in the form of quick response mag-shield drones, that shoot out into the direction of an unexpected radiation beam, could be the difference between 0.1 seconds of sickening radiation and death.
What is the relationship between mag-shield drones and LCPBs?
The same concept can be used at even greater distances. The magnetic field will not be powerful enough to promptly ionize heavy particle beams approaching far below the speed of light, but it can work use its field to hold in a bubble of plasma. LCPB particles traversing a plasma can be quickly ionized, allowing them to be affected in turn by the magnetic field.
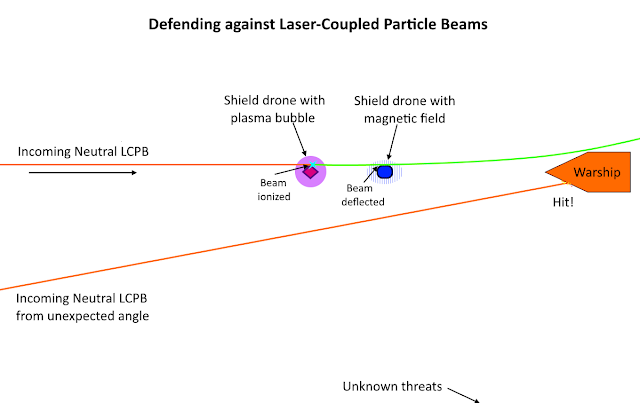
The further away the mag-shield drones are from the main ship, the weaker and lighter they can be, but also protect it from fewer directions.
Counter-beaming is another option. A LCPB’s arrival will be announced by a guide laser to give several seconds of warning at longer ranges. The targeted ship could aim down the beam path with its own laser. This ‘counter-LCPB’ beam would have an intensity profile opposite to that of a gaussian beam: weak in the center and intense at the edges. It would use the same Laser Coupling technique to push particles out of the center of the beam and therefore increase divergence.
What is the relationship between mag-shield drones and LCPBs?
The same concept can be used at even greater distances. The magnetic field will not be powerful enough to promptly ionize heavy particle beams approaching far below the speed of light, but it can work use its field to hold in a bubble of plasma. LCPB particles traversing a plasma can be quickly ionized, allowing them to be affected in turn by the magnetic field.
The further away the mag-shield drones are from the main ship, the weaker and lighter they can be, but also protect it from fewer directions.
Counter-beaming is another option. A LCPB’s arrival will be announced by a guide laser to give several seconds of warning at longer ranges. The targeted ship could aim down the beam path with its own laser. This ‘counter-LCPB’ beam would have an intensity profile opposite to that of a gaussian beam: weak in the center and intense at the edges. It would use the same Laser Coupling technique to push particles out of the center of the beam and therefore increase divergence.
C-LCPBs will have a tough time as they have a narrow window of opportunity to get themselves aligned as well as possible with the incoming LCPB. They can never be perfectly parallel with the incoming beam, so they are less efficient. Worse, their need for large lenses makes them hard to protect when they become targets themselves.
From a visual perspective, battles involving LCPBs are more interesting than laser-dominated combat.
The neutralization step emits a small bit of light at the beginning of the particle beam's journey. Its guide laser will leave a dim trail of scattered photons from where it interacts with the particle beam. A brighter flash will occur where an ionizing laser meets an incoming LCPB, with the most spectacular sight being the interaction with a plasma bubble. C-LPBs have to make up for their reduced efficiency by using more power. This power ends up scattered as photons anyone can see.
This was prepared with the gracious help of GerritB, Kerr and Imallett.
From a visual perspective, battles involving LCPBs are more interesting than laser-dominated combat.
The neutralization step emits a small bit of light at the beginning of the particle beam's journey. Its guide laser will leave a dim trail of scattered photons from where it interacts with the particle beam. A brighter flash will occur where an ionizing laser meets an incoming LCPB, with the most spectacular sight being the interaction with a plasma bubble. C-LPBs have to make up for their reduced efficiency by using more power. This power ends up scattered as photons anyone can see.
This was prepared with the gracious help of GerritB, Kerr and Imallett.
Excellent work MatterBeam! Now I have to figure out how to save my worldships...
ReplyDeleteThanks! It always ends up being longer and taking longer than I expect, but I hope the outcome makes up for it.
DeleteWorldships are going to be tough to protect. Their best bet is extreme redundancy and survivability as well as an 'expeditionary force' the brings the fight to the enemy and far away from the worldship.
No matter how long it takes, thank you for putting in the time. I can't tell you how much I've enjoyed reading over this website and re-thinking everything along the way. Keep up the fantastic work!
DeleteNo need to worry about how long it takes, good work can't be rushed, and you do great work.
DeleteI've asked in more detail over on the discord, but the km thick iron fuel tanks you figured out for a decent amount of delta-v would certainly give some survivability. I think the main part of my ship's defences will end up being from their laser weapon web, and indeed bringing the fight to the enemy. Once the enemy gets close, though, those ships are at real risk. Good for narrative.
Both of you, it is much appreciated.
DeleteI'll respond in detail to your worldship question tomorrow...
No problems MatterBeam :)
DeleteHuh. I just realized that if you built the turret for one of these things right, it could actually look an awful lot like the 'death rays' on alien spaceships in old retro-sci-fi. By this I'm referring to those ones that look like a dish antenna with a rigid string of balls stuck through the middle. The dish is the laser mirror, the central rod is the particle accelerator.
ReplyDeleteAlso worth noting is that a high-powered guidance laser would also have the side advantage of contributing a bit of damage in its own right, even if that's really not the point.
Quite right. Did you write the same comment on the SV forums?
DeleteAs for your latter point: That's possible, yes.
First of all i am glad to know you are continuing your blogs, you are one of the best.
ReplyDeleteThis blog is as detailed as any other but i am overwhelmed of knowledge.
I think you use the tractor beam tech to focus your partical beam. This should increase range and penetration.
Under what circumstances will a military use this than just a bigger partical acclerator or a high speed mac cannon.
Also i want some numbers. How would a 5GW acclerator fair against aero gel, carbyne and steel armore at 1000km,10000km.
The blog lives on! Thanks for the kind words.
DeleteTractor beam technology is sadly fictional. 'Optical Tweezers' are the closest non-fiction example.
The military will use this if it wants weapons that can strike targets across millions of kilometres of distance. No other weapon I know of can do the same without having to be many thousands of times bigger and heavier.
A 5 GW LCPB could focus its energy in a tight circle about 0.2 mm in diameter at 1000 km and 2 mm in diameter at 10,000 km. It can burn through several kilometres of any material, even carbine, every second. No spaceship is that big. Therefore, these beams will act to poke very long, very thin holes through targets.
Finally, laser fortress can't rule unchallenged. That's a good news. :D
ReplyDeleteCan the functions of bubble drone and field drone be put together into a single drone?
Having two drones means taking up more spaces and payload, after all.
That should be possible, but the risk is that the particle beam will plough through the plasma bubble without fully ionizing.
DeleteJust thinking about defenses, if a vessel turned tail and did a hard burn away from the target, would the gas cloud and the velocity of it's particles confer any significant protection? It would be inconsequential as armor as the gas doesn't have any structural integrity but it could cause some beam divergence. The earlier you fired the drive the more protection you might get. Just thinking of a last ditch effort.
ReplyDeleteThe other possibility is if you're using an atomic drive, the shielding for the drive system itself should protect to an extent. Then you'd have the problem of the PB burning through your drive and possibly your neutron shield but if the drive is killed by the beam you don't need the neutron shield after that. Again, last ditch effort.
The problem with relying on that effect is that rocket engines want to reduce the density of their exhaust as much as possible before it exits the nozzle.
DeleteThe heaviest particle beams would have the lowest penetration (millimetres through carbon at 2300kg/m^3). If the exhaust right at the exit of the nozzle is a modest 100 Pascals and composed of room temperature hydrogen, it would have a density of just about 0.00004kg/m^3. The particles will pass through it very easily (penetration several kilometers!).
If you increase the pressure and reduce the temperature so that there is more gas in the way, you will find your propulsion performance dropping to abysmal levels!
The best way to defend against an LCPB beam is to rotate the armor. If you continuously put new armor in front of it, it will have to start burning from the surface again and again, creating wide and shallow grooves instead of deep holes.
GWs of power at 2mm at 10000km.(deep breathing...) Its a total overpower, i think ODPs would be the first to have them.
ReplyDeleteCould you explain more about optical tweezers because lasers them selves decepitate at thousands of km how could they be work at millions?
For defence i dont think rotating your armore would work, just the raw power of LCPB previously what was a hole has now cut your ship in halves.
LCPB is good at focusing lets use that aginst it... If you ships are made simple and redundant enough you can shrug off multiple holes . Even better if self sealing materials are used (like in fuel tanks).
BTW this is of topic but just by noticing the flawlessness of all your posts makes me wonder, are you just one guy our you have a "development team". Tell me in am underestimating you.
Yep, they're pretty powerful when they hit. What are ODPs?
ReplyDeleteThe laser should be imagined as a sort of barrel. A 1000km long barrel that helps put particles on the right track. Just like a bullet doesn't need further aiming after it exits a barrel, the particle beam continues travelling through space until it hits a target.
For defense, the armor can be rotated so fast that the beam's energy is spread over its entire perimeter. If the target is 10m wide, it has a circular perimeter of 31.42 meters. The 2mm wide spot would have its energy spread over a band 2mm tall and 31.42 meters wide... this is a 15,710x reduction in energy per area.
These topics take a lot of research and consulting really smart people, I just hope that when I put it all together is makes sense, isn't too complicated and stays interesting. Development team is 1 person's free time!
ODP is a short form of Orbital Defence Platform, if Unknown is using the same term from Halo Series
DeleteThanks.
DeleteAmazing as always.
ReplyDeleteTrying to figure out the various permutations and see where the various "lines" intersect. I still believe the classic Ravening Beam of Death (RBoD) capable of burning though metals, ceramics and carbon fibre has the edge in most space battle scenarios. Since you can deliver effects out to one light second away, you can strike targets before they can strike back with even the "pencil beam", while the ship carrying the RBoD can certainly move an appreciable distance if you are attempting to engage from beyond one light second. It will always be in the interest of the RBoD to engage at the maximum distance possible, and even at one light minute you are still in "scorch" range.
The ion beam armed opponent can have a flickering spiral of beams to attempt to clip the RBoD (and the all important diffraction grating they use to focus an x-ray laser beam), forcing the RBod to continue moving during an engagement. This is likely true if the RBoD is a self powered warship in a constellation or a focusing element at the end of a laser web.
Of course the problem is when you want to move in closer than one light second. Now as you close in the time lag drops and hit probability rises for the ion beam, and the RBoD has much lower chances of success, or even survival.
In any sensible organization, there will be a multiplicity of different vehicles for mutual support and overlapping effects. If I am reading these posts and understanding them correctly, the ion beam is a wonderful mid range weapon to shield the constellation as part of an overlapping layer of effects, covering between Laser Stars and point defense Fire Stars unloading their CASABA Howitzer rounds at incoming Soda Cans of Death (SCoDs).
Adding to the fun, of course is the constellation itself might be riding to battle on a huge ion beam from the home planet, which can be refocused and repurposed when the constellation "casts free".
Every post just makes the future far more interesting and complicated...
And thats what makes things fun!
DeleteI can envisage the following situation:
-Laser forts counter laser fleets by having much more range.
-LCPB fleets counter laser forts by having much more range.
-Laser fleets counter LCPB fleets by having lightspeed weapons.
It's not really a triangle (unless the attacking fleet comes along with its own laser fort) but it does give room for both lasers and particle beams to have a role.
Mix in stealth ships, kinetic impactors and Casaba Howitzer rounds and you'd got something that looks more like a nonogram than a triangle!
Personal preference aside(since I am pro-ship and I find some fort ideas seem to be kind of "against the trend simply for the sake of against it"), laser forts can't rule unchallenged is a good thing.
DeleteThey have their uses, yet to me, sometimes they are overrated.
And am I the only one find that laser fort rulez is the return of "ICBM rulez and other forces go home"?
Many SF authors have fallen into the simplifying assumption that one weapon system will be better than all the others. It is bad for the setting they create...
DeleteYes i was referring to orbital defense platforms.
ReplyDeleteI wanna know is optical tweezing possible with solid pellits, bebecausi dont want big shiny ion cannons they just look too fictional. I prefer microscopic pellets fired electrostaticaly.
Rotating the ship might help but your crew wont be that happy. Also do your know any ship design which uses spin gravity still looks good othere than OPAS Behemoth.
Your solid pellets would have to be small glass beads. Those beads would bend light, and therefore can produce the optical tweezer effect as described here: https://en.wikipedia.org/wiki/Optical_tweezers#Ray_optics
DeleteThe problem is that it is very hard to accelerate glass or crystal beads to a high velocity. Even with perfect aim, it will take them a long time to cross the big distances separate spaceships during a battle.
I don't know about what rotating ring designs are out there.
I ment "because i dont.....
ReplyDeleteWhy not use a laser with shoter wave length they will pass through solids also you can focus them for longer.
ReplyDeleteAlso wouldn't a back partical shadow the laser for the front particals, like i dont know but particals beam should be dence right?
I find it hard to understand your comment with the spelling mistakes (particle?) but to my best guess:
Delete-Lasers are absorbed very quickly in solid matter. Even the shortest wavelengths, like gamma rays, only get through 3cm of lead before being reduced by 94% in power.
-The particles do not cast a 'shadow' as they are smaller than the wavelength of the laser. It is not a problem.
Is LCPB prone to all laser problems.
ReplyDeleteYes, there is a direct relationship between drive power and the beam weapon.
DeleteI think LCPBs range is the biggest killer to it. Just the possibility of where the enemy can be is to much.
DeleteI think this can be tackled if the beam can move, i dont know much about optical tweezers but if the ions are attached to the highest intensity of the laser then can we make one side of the laser more intense to move the ion beam towards that side.
Once again, I'm incredibly impressed with your research and article.
ReplyDeleteBased on your studies, can you summarize the scenarios where: casaba howitzers, kinetics, lasers, lcpbs and missiles are best used?
Thank You,
No, thank you!
DeleteThe use cases for each of those technologies depends mostly on their relative level of development and effectiveness. If they are somehow all equal at a certain point in time, I would foresee the following:
-Lasers for long range attacks against moving targets, as part of Laser Weapon Webs with uncapped range, striking ground targets and to defeat anti-particle beam defenses.
-LCPBs for long range attacks against slow/stationary targets, short ranged attacks against moving targets, and for defeating anti-laser armor.
-Kinetics: uncapped range against stationary targets, or to deliver a constant stream of 'sand' to hinder laser weapons.
-Missiles: variable range against moving targets depending on closing speed, works best when using stand-off warheads.
-Casaba Howitzers: warheads for missiles, siege weapons that can break an asteroid fort in one shot, or a defensive measure against incoming missiles/kinetics.
I hope this is what you were looking for.
Absolutely! Thank you very much, M.B. Would you see 5 types of specialized attack platforms, or would it be optimal to combine some of them in the same attack platform, e.g., lasers + LCPBs? (I would assume it would be inefficient or impractical to have all five in one.)
DeleteIt is too situational to give a good answer.
DeleteA rational arrangement is to have on one side the biggest possible weapon of the type dominant at the a time, paired with a counter-counter weapon, and have the biggest possible counter-weapon to defeat the dominant type, paired with a counter-counter-counter weapon.
For example, we could have lasers be the most effective weapon in terms of output and effectiveness. The counter-weapon would be pulsed lasers designed to destroy mirror optics. A counter-counter weapon would be sand-stream kinetics and the counter-counter-counter to that might be some sort of transparent whipple shield.
On one side, there would be a giant laser backed up by sand-stream kinetics. On the other, a pulsed laser backed up by the transparent whipple shields.
I tend to believe that the rocket equation would drive every platform to be as specialized as possible. Since "every gram counts", carrying anything "extra" will degrade the performance of the carrier vessel. Since we are talking about military spacecraft, they will all need to be self propelled at the moment of contact (using beam power to get to and from the Hill Sphere of operation is useful for reducing mass and the need to carry lots of propellant, but being stuck to the beam will limit your options considerably.
ReplyDeleteThis means each element in the constellation will be an "eggshell with a hammer" almost by necessity. Multi gigawatt Laser Stars for long range firepower. Fat, missile bus Kinetic Stars to fill the sky with thousands of SCoDs to overwhelm enemy lasers by sheer numbers. Fire Stars based on Kinetic Stars but carrying nuclear CASABA Howitzer rounds for close in defense. And now the medium range particle beam weapons for mid range coverage (Beam stars?).
What I don't think we'll see are frigate like general purpose ships with a brace of different types of weapons, simply because it will be overmatched at any range by the corresponding platform in the enemy constellation
Correct on all counts. You get my meaning!
DeleteSecond Tier powers might have to have more generalised ships simply to get enough ships out there to be effective. Even now, the Royal Navy's only truly specialised ships are the aircraft carriers. We simply cannot afford to man a whole fleet of AA only, ASW only etc ships.
DeleteCosts and manning issues (even if automated, some "ground crew" may be needed), may mean that "frigates" are used by 2nd rate powers that fear each other more than the local superpower.
Specialised ships may also be only acceptable if that particular weapon is flexible. A missile armed stealth carrier can use different warheads and different sized missiles for each situation. A laserstar can accelerate kinetics via light-craft propulsion. A single main weapon that is flexible reduces the need for many ships with their expensive engines to accompany each other.
Cost and manning matters. The rocket equation isn't the only tyrant out there.
Good points. When I think of specialized ships, I actually imagine the Russian Navy equivalent in space - focused on defeating generalist opponents with maximal cost effectiveness tactics.
DeleteIt might end up being a U curve. On one end, huge budget allow you to have very high performance, multi-purpose ships. On the other end, budget restrictions means you need to make the most of each ship, so you add in multiple functions by necessity.
A particle beam can be used to accelerate kinetics, even more effectively than a large can.
Would a target of a LCPB receive forewarning of an attack by detecting the coupling laser before the beam strikes? There is a lead difference, but in some cases where there is little lead (e.g. if the target is travelling head-on towards the attacker or they just aren't moving relative to the attacker) the laser will still hit them first. Is this a legitimate weakness?
ReplyDeleteYou are correct. It is a weakness. It gives the target a fraction of a second of warning in most cases.
DeleteHow would the Pellet Gun of Doom (PGoD) fit into all of this?
ReplyDeleteIt retains its strengths, such as infinite range and the ability to physically destroy armor without having to heat it up, but now it has to compete with a weapon that travels even faster and is just as efficient.
DeleteInteresting...are there any ways for the PGoD to compete? Perhaps as "anti amour" weapon to destroy armour and then bring a RBoD or LCPB?
DeleteAt ranges where the intensity of the particle beam is not enough to melt or vaporize armor, especially when active cooling and sloping is involved, PGoD is very effective.
DeleteSo in short, such a setting would have PGoDs for general, but mostly, long range and anti shield(?) drone combat, LCPBs for mid range and stationary target engagements, and lasers for...pretty much everything else. I don't see a way that missiles can compete (unless they are Casaba Howitzers of course) and the small 'fighter' ships you suggested in the PGoD post will definitely have a hard time completing there mission (what ever it is).
ReplyDeleteAlso, how advanced do you have to be to have all these weapons? is the pare minimum advanced fission? Or do you need fusion for all of this?
And lastly, would a droplet radiator be viable on a combat ship? And if not, what is better than a solid radiator on a combat ship?
Things change slightly when you realize that kinetics can be accelerated by the same long-ranged beams to become very high velocity projectiles...
DeleteThese weapons do all exist in one form or another on Earth, in laboratories today already. Their energy source is electricity, and electricity can be made in so many different ways, including burning fuel to solar heating and the different types of nuclear power.
A droplet radiator won't really work on a space warship that always need to be moving, but you can adapt it for combat by fitting it inside a large balloon that lets heat out but traps droplets inside. If you want the best of liquid droplet and solid radiators, I suggest you look at the wire radiator designs I wrote about in the post 'All the Radiators'.
Thank you!
ReplyDeleteSo what you are saying is that you can accelerate or boost a missile via a LCPB or laser? That would actually by rather interesting. How would this look though? would you have tubes in the front of the ship to minimise surface area? Or would someone go full broad side and use the same LCPB or lasers of point defence as well. Perhaps you boost the missiles and then have a on board rocket to propel it and cause random 'jinx' movements to avoid defences? or is stealth the better option. And what war head would they use (asides from Casaba Howitzers), kinetic, sand, nukes?(doubt it)
How would laser web ways fit into all of the? PGoDs would just obliterate any mirror that it hit, (and missiles...) meaning that you would need a self proplled fleet of ships to protect the relays. Or just have Hydrogen streamers near the enemy planet for first strike capability (then how is there a war after both sides have been nuked?).
And would the fleets of independent ships (if there are any) be arranged in the Laser, Fire, Kinetic etc Stars system that appears to popular on this forum (would PGoD be...Uh...Pellet...Stars? That's lame) or would they more than one function? (Perhaps economics are something to consider?)
Also, are the "fighters" of the PGoD post possible in such a setting? I mean, in that post, you were assuming that the PGoD would rain supreme. But with laser webs, Howitzers, and LCBPs all over the place, I am left scratching my head on how the "fighters" could compete.
And lastly, to get this interesting but most likely improbable idea out of my head, is it possible to make a torchship from a nuclear gas core engine. We are already coming up with nuclear engines that have torch like qualities. But I feel that an actual fission torchship is asking for to much. and is it ridiculous to have a setting where fusion is available but is to big to fit on ships and thus fission is the better option?
P.S. Sorry if I am being a bit too...naggy, So many questions in my head!
No worries! I love answering these questions.
ReplyDeleteYou would accelerate the missiles from outside the effective range of your beam, or else you'd be using it as a weapon directly. So, for example, if you can deal damage at 100,000km, you'd start shooting missiles from 300,000km away.
These distances gives you plenty of time to accelerate the missiles to very high speeds. You can simply drop the missiles behind you and have them use their maneuvering thrusters to position themselves in front of your beam one by one. With beamed propulsion systems, you can get high Isp and high thrust. This means you can quickly accelerate missiles to high speeds. By staggering the launch velocities, you can get many missiles accelerated in series to converge onto a single strike on the target.
Stealth techniques definitely help the missiles cross the large distances between beam-equipped ships. Sudden maneuvers in the terminal phase of their flight can help avoid interceptors.
If you know that a large number of missiles coming at you at high velocity will pop up on your sensors at relatively short distances, then you can't just have a simple warship equipped with a huge beam weapon survive for long. It would need kinetic interceptors, anti-kinetic armor, even anti-missile missiles. Its best anti-missile weapon is its giant beam, but it would have to prioritize between shooting down missiles endlessly and trying to hit its enemy, perhaps with its own missiles. There are a lot of moving parts here, with plenty of choices to make regarding optimization.
A laser weapon web is another layer of complexity on top of everything. It greatly increases beam ranges, but also means missiles can be accelerated from farther out and become gigantic missile waves that can overwhelm any sort of defense. Part of the missile wave could be more mirror relays, either as a second stage of acceleration for missiles or to shoot down incoming anti-missile missiles... it's quite fun to think about.
Hydrogen steamers are not invulnerable. A planet that suspects their presence can spend months scouring the sky for the slightest trace of them, and pre-aim huge ground-based lasers to smother their general position with energy to detect and/or destroy them the moment they slip up and reveal themselves.
Ideally, a fleet is composed of ships that can break all types of defense and handle any sort of offense. A pellet streamer, laser beamer and particle sniper would join a missile arsenal, mirror web deployer and phased array interptor to become all-rounded in effectiveness. In reality, you are most likely going to have one aspect more powerful than all others (for example, superbly long ranged LCPBs) and several holes in the defensive capability (like no anti-missile missiles). Fleet commanders must work to their strengths and avoid facing their weaknesses.
Fighters still work when LCPBs are involved, if you just increase the distances which you consider short range! I consider a 'fighter' to be a smaller craft with a shorter ranged weapon that gets close thanks to its smaller cross-section and ability to dodge weapons.
For example, a 2m wide ship will only be hit 1/50 times with a 0.5C beam weapon at 200,000km if it accelerates at just 2m/s^2. It can afford to have 50x less armor than a 14m wide ship accelerating at the same rate, or 10m wide ship that only manages to sustain 1m/s^2.
Gas core reactors are pretty impressive. Their Isp of 2000s without radiators means that with a mass ratio of 5, that fighter in the previous example can sustain their acceleration for a whole 4.4 hours. They can increase their Isp to 5000s with radiators, and even further if you manage to create an electromagnetic confinement system for the uranium plasma. An interplanetary ship with a mass ratio of 10 would be able to zip to Mars in 11.3 days at the closest approach, 46.5 days if it had an Isp of 5000s.
So what who are saying, is that gas core engines are 1G Torchships? Neat.
ReplyDeleteSo I’ve been wondering. How big are they. How would these ships look? I know that the Stealth ship (dark star) would look like a tube in a tube; but how all the other ships look? Missile busses looking like great leviathan (ie fat whales) with a LCPB at the front while missiles unload from the back waiting there turn to accelerate (the way you describe it makes it sound like artillery). Great swords of pellet ships and laser stars with quivers (space aircraft carriers?) loosing fighting daggers into space?
How would the laser/pellet/particle weapons be protected? Would the weapons be keel mounted or would they turreted or something in between?
What would AAA Defense look like? Lasers dividing their beams, miniature pellet guns, or the active protection armour that would make some parts of the ship look scales.
And lastly, would the cold particle beams used (since everyone seems to be gushing over the LCPB)
This is all with wires radiators( how much heat do they radiate?)
Gas core engines as we know them allow for rapid interplanetary travel, but they're not really 'torchships' because they don't allow for continuous high acceleration. This doesn't exclude future advances in uranium plasma technology that turns them into true torchships.
DeleteFor example, gas core engines with 1MW/kg power density and let's say, 10,000s Isp, representing 20% of a spaceship's dry mass and loaded with enough propellant for a mass ratio of 5 would be able to accelerate at 0.14g on average for 32.5 hours in total and accumulate 157.8km/s of deltaV. That allows for interplanetary travel at 78.9km/s, which is... very fast!
If we go even higher, with 10MW/kg engines and 25,000s Isp, we could get 0.28g acceleration for 40 hours and a total of 394.7km/s. That's proper torchship performance!
How ships look depends on your technological assumption and the specifics of the setting.
That said, I could expect missile carriers to be nothing more than stacks of tubes, like a truck carrying logs, that ferry ammunition to a beam-equipped craft. The laser or particle beam warship has a sharp cone of armor that allows it get close to targets if necessary. There is no real need for it to have internal ammunition stores if the missiles are only being accelerated from outside beam ranges. It is very much like artillery, as you say.
At very long distances, you only need to move your nose by a few degrees to catch any and all targets within your firing line. All big beam weapons would be spinal-mounted, with lenses making the tiny adjustments needed for aiming. You would want shutters to close your lens from counter-fire or kinetic attack too.
Anti-missile/projectile defense would be done most of the time by you main beam weapon. As they get closer, you can split up you laser beam into multiple turrets that have an easier time tracking the projectiles. Similarly, RF generators could divert their energy to powering shorter, lower energy defensive particle beams.
You would never want to use kinetic impactors like pellet guns to intercept energy projectiles, as it is extremely wasteful. If you want to stop something dead in its tracks, put a net, plate or stack of lightweight foils in its path - this is what I mean by interceptor drones.
LCPBs are a bit more advanced technology, but they would only be useful at extreme ranges and would likely not be flexible enough for defensive use.
Wire radiators are an option if you want better kW/kg values for your waste heat management system than flat panels but find droplet radiators unsuited for the rapid accelerations during combat. They'd look like spider silk webs glowing red to orange, trailing behind the spaceship.
Wouldn’t the radiators get caught up in the exhaust? (Making humped armour to hide the wires more viable)
ReplyDeleteHow big would the crews and the ship be? Assuming there are gas core torches, it would really depend on automation, I assume?
Also, could you point out some sources to help with establishing the technology of the setting.
P.S you make a post dedicated to drones:)
Well, it would be reasonable to assume that the radiator wires don't extend past the end of the nozzle then!
DeleteThe ships will be sized according to the needs of the mission, and the crew sized do indeed depend on automation.
It is the author or worldbuilder who decides the technology of a setting. There is no 'correct' answer for this.
Well, thank you for your answer. But it appears that a question of mine was deleted for some reason. It was if you could point to some sources that could help with setting and technology since everyone says all these ideas but rarely goes into the effects of say a new technology.
ReplyDeleteI know a few sources already, just wondering if you know any others.
Sorry, just realized the earlier question did have my query, just not in detail.
DeleteHello Matter Beam, et al,
ReplyDeleteWould the following scenario be logical/feasible?
Very few military ships/"stars" but lots of autonomous weapon systems (AWS):
A number of factories/beam-launch facilities manufacture and launch large quantities of various weapons (PG, Casaba, kinetic, laser, LCPB) with AI and moderate-maneuverability capabilities and optimally deploy them.
At what distances could these various types of weapons be detected prior to being used?
Keith
If they are optimally deployed, then they are predictably deployed. This means that it is somewhat easy to narrow down or even pinpoint the location of AWSs by using the collected data over days, weeks, or months of observation. This is assuming that they have really good stealth.
DeleteThen, with that location information, the defenders will pre-position countermeasures. These can be big lasers, missiles, big guns and so on. The moment a war is declared, you activate your AWSs and they fire their countermeasures.
The battles will be conducted using the survivors of that initial rush to action. If you managed to sneak by a few AWSs in non-optimal positions, or if they got enough of their own weapons to avoid your attacks, then you are able to move against targets that do not have their defenses already set up.
Thank you again, M B. This sounds to me more like a Cold War Triad/MAD-scenarios than a naval battle-one.
DeleteCheers,
Keith
How does a laser coupled system function when using quasi neutral particle beams(overall neutral charge), can the laser still be used?
ReplyDeleteYes... so long as the laser is intense enough to overcome the thermal motion of the particles. There is an upper limit to this intensity - too much and the particles would ionize and repulse each other. So, you are restricted to cold particles.
DeleteHow much energy do you need for the particle beam weapon to be use to attack target (and kill with radiation, the crew or electronics) on the ground on earth ?
ReplyDeleteIt is a complicated question, but I would say in excess of 1 PeV.
DeleteHalf of that energy, 450 TeV, is still not enough to penetrate the atmosphere (https://physicsworld.com/a/cosmic-gamma-ray-energy-record-shattered-by-high-altitude-observatory/).
Why so much energy ? The atmosphere is 10 tonnes/m2 or 1000g/cm2. With your penetration formula, the penetration for a 3 GeV proton beam is 3715g/cm2 more than the atmopshere.
DeleteI'm wondering what kind of practical range we could get out of this kind of technology.
ReplyDeleteI've been looking into magsails and have found out that there's a few interesting aspects of them that give them advantages over laser powered photon sails when powered by high power particle beams. There are also plasma augmented magsails, massively increasing the potential area of the sail. So since this technique reduces the divergence immensely I was wondering how it might apply to high powered magsail vehicles. To me this lifts the performance limitations to some extent, the primary one being the effective range of the particle beam. If this concept can improve that effective range then higher mission delta-v may be possible, enabling rapid interplanetary transport and fairly high speed interstellar vehicles - provided the magsail can still slow down from such high velocities at a reasonable acceleration in the interstellar medium.
The range depends on the scale of the device and the coupling duration. A relativistic proton beam guided by a laser beam could maintain its initial radius for millions of kilometers. Just coupling the two for a single minute could get you 18 million km before the beam starts diverging again. One hour and you get a billion km!
DeleteBecause the beam does not diverge within that time period, you don't need features which increase the size of the magsails.
The problem is, I have been discussing with a physicist the possibilities of this beam. While the PROCSIMA study has moved to Phase II of NASA's NIAC program and we hope to get a proper white paper on the concept, we are worried that the interplanetary medium will interfere with the beam sooner than later. It might not be possible to couple a laser and particle beam for an hour, or even a minute, unless you fire it in very 'clean' space you might only find at the edge of the Solar System. Remember that the Sun is pumping out solar wind and filling interplanetary space with about 5 to 100 particles per cm^3. In mass, this is 8*10^-21kg/m^3 to 1.6*10^-19kg/m^3. Not much, but it could be enough to disturb the beam over long ranges.
Given the absurdly tiny radius of the LCPB at small distances (like 2cm at 100,000km), resulting in stabbing clean through enemy warships with little needle holes, might it be possible to deliberately not turn on the laser if a relatively close ship wanders into the right place, so as to do the usual massive damage to a decently large area, rather than just sticking a pin through the target?
ReplyDeleteObviously it's not going to be a massively frequent occurrence, given that these LCPB ships would be huge and not exactly in position to deftly manoeuvre, but if you have a 0.5c beam at a range of a bit under a light second or so, you've a pretty damn good shot and the wider burst might do more damage - that is assuming of course the lower intensity is still enough to burn through their armour in the first place.
With very powerful LCPBs, you could finally recreate the scifi trope of beams slicing through spaceships. Making circular movements with the beam can remove armor and internal structures from spaceships very efficiently, at least when compared to having to melt that same armor or structures.
DeleteAh of course! I hadn't thought of that; the beam can just be moved around, and then there's the fact the ship being shot at is moving as well... So in fact if you do just point slightly ahead of the ship, and the beam arrives just in time to hit the nose of the ship, with a long enough pulse that it lasts approx 1 ship length, you might very well cut the whole thing in half!
DeleteThanks for the swift response!
And also, just thanks for going over all this stuff in general. I love my sci-fi worldbuilding but always want my equations to work in some way, so it's amazing to have such a detailed resource! Sometimes I find that somewhere like Project Rho is a bit of an overload (the conventional weapons page particularly is... long), but the way you have everything laid out on this site is great, with the focused articles. Also,combined with your other particle beam article with its equations, I finally have a justification for having a 500m long Dreadnought with an absurdly high powered and high velocity spinal mount particle beam, so that's also good.
I'm glad to hear that. Good luck with your projects and feel free to ask more questions here!
DeleteThis comment has been removed by the author.
ReplyDeleteWouldn't that also allow particle beam in atmosphere ? With these 2 laser profiles in succession: 1- max-low-max, Ionize atmosphere and push the ion on the side to create a void tunnel, laser induced acceleration must compensate the air particle acceleration due to atmospheric pressure. 2- max-low-max-low-max. the center low-max-low is the classical coupling guiding the particle beam. The sides max, maintain the atmospheric ionization and side pushing to maintain the void.
ReplyDeleteYep, it would work to remove the atmosphere in the path of the beam, however you wouldn't get a 'cold' beam at the end.
DeleteAre there any requirements for the coupling laser or any laser that get enough power could do the trick?
ReplyDeleteThere must be some requirements for laser intensity and intensity gradient, but I couldn't easily find it in the few pieces of literature available on this concept.
DeleteIf you keep pointing the laser at the target, shouldn’t the particle beam go with it? In that case, it kind of acts like a light speed weapon, because it will hit wherever the laser is pointing, right? So it would be able to completely beat lasers. I’m sorry if I overlooked something and this is a bad question.
ReplyDeleteIs it possible for the LCPBs to be diverted? Like, for lasers you can use mirror drones to divert the beam and with particle beams you can use magnetic fields to move it around, but I can imagine it would be very difficult to get the laser and particle beam lined up again, consistently, and in battle conditions no less.
ReplyDeleteI'm not sure there is any mechanism to divert both the laser and particle beam with such precision that their internal gradients remain matched!
DeleteAlso, magnetic fields work on charged particles!
thanks you
ReplyDelete